Gabriel Ortega: “How Do Halophiles Endure Extreme Salinity?”
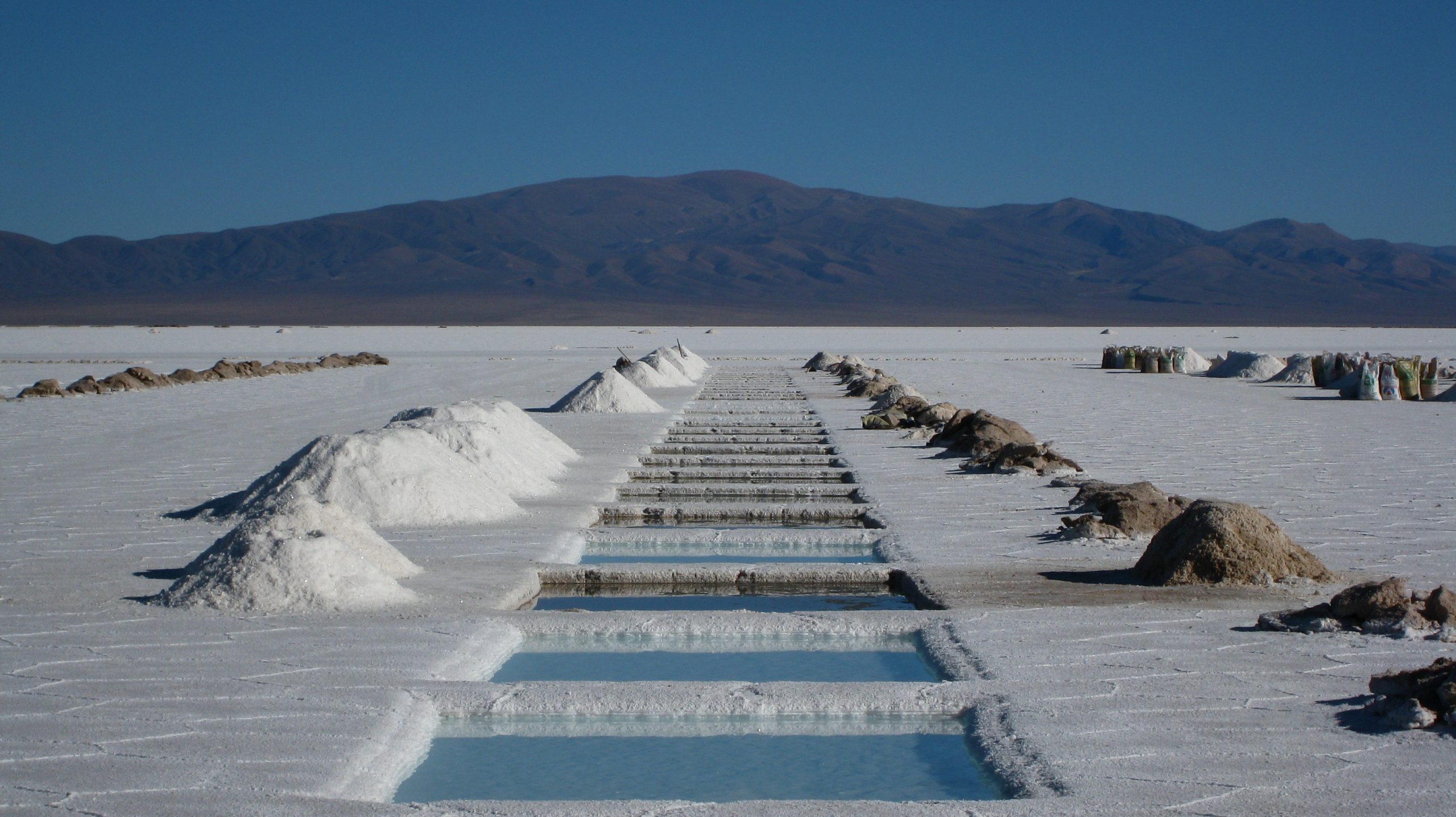
The slow but unstoppable progress of evolution has enabled, step by step, mutation after mutation, the conquest of virtually all ecosystems on Earth. Even in those places where conditions are far from “standard”, where the presence of organisms seems unthinkable, life has found its way. These small conquerors are called extremophiles. Thus we find thermophilic organisms that survive at high temperatures, or their psychrophilic cousins surviving at low temperatures, or organisms that adapted to the absence of oxygen, to extremely acidic media, to high pressure… Of all these, we highlight the organisms that adapted to extreme salinity: halophilic bacteria and archaea.
The first news of halophilic organisms are found back in the ’30s in the Dead Sea, so named because it was believed that their high salt concentrations prevented the presence of life in its waters. As it turns out, ironically, there is life in the Dead Sea. And I do not mean to tourists photographed floating on its dense waters, but unicellular microorganisms that have been able to adapt to osmotic stress, living in a virtually salt-saturated aqueous medium. The high salt concentration exerts an osmotic pressure that squeezes and dries non-adapted organisms. Halophiles offset this negative osmotic pressure by increasing the intracellular concentration of solutes. Specifically, extreme halophilic organisms that survive salt concentrations up to 4 M accumulate molar concentrations of potassium chloride in their cytoplasm.
The more astute readers will have noticed that salt is a bad travel companion on biological environments as it destabilizes and causes irreversible aggregation of the constituent proteins. So, how do halophilic organisms adapt for maintaining a stable and functional intracellular machinery? It has been known for some time that proteins belonging to halophilic organisms are virtually identical to those of other organisms. However, their amino acid composition is markedly different (Figure 1). On the one hand, there is an abundance of acidic residues on the surface, as well as short and polar side chains. Furthermore, the number of amino acids with bulky and hydrophobic side chains is significantly reduced -particularly lysines with its long, disordered side chain. Consequently, halophilic proteins are very stable at high salt concentrations, while maintaining its solubility and functionality.
Figure 1: Relative abundance of each type of amino acid in halophilic proteins over its natural abundance in other proteins.
In a paper recently published in the journal Chemistry and Biology, Ortega et al. study the relationship between amino acid composition and haloadaptation. Using molecular biology tools, they replicate in vitro the evolutionary process and design systems of study with different degrees of haloadaptation. By applying biophysical methods and atomic-resolution structural techniques (nuclear magnetic resonance spectroscopy) they have conducted a comprehensive characterization of folding and unfolding states of halophilic and non-halophilic proteins under conditions simulating those of hypersaline environments. Thanks to this study, they have managed to unravel the mechanisms linking amino acid composition with the different contributions to the stability of proteins, as well as the interaction between protein, solvent and cosolute. The action of two opposite but complementary mechanisms confers great stability to halophilic proteins in the presence of high amounts of salt: the interactions between the abundant negatively charged residues on the protein surface and the potassium ions in solution (positively charged) stabilize the folded state, while the increase in polar surface (and the decrease in hydrophobic area) favors the preferential hydration of halophilic unfolded states, which in turn increases the preferential exclusion of ions from the surface and consequently destabilizes the unfolded state. So, we have two opposite mechanisms: preferential interaction with ions in the folded state against preferential exclusion in the unfolded state. However, the effect of both mechanisms goes in the same direction, stabilizing the folded (native) form of halophilic proteins in the presence of salt (Figure 2).
Figure 2: Two opposite but complementary mechanisms: electrostatic interaction in the folded state and preferential exclusion of ions in the unfolded state. Both have been evolutionarily optimized by amino acid selection and cooperate to populate the native form of the protein in high salinity environments.
This study not only answers an interesting biological question, but also highlights the mechanisms involved in the behavior of macromolecule-solvent-cosolute ternary systems. Their findings may be useful in other fields: Hofmeister series, the effect of other non-ionic cosolutes, excluded volume, protein folding, organic solvent mixtures in bioreactors, agriculture in areas with water shortages… There are also exciting consequences from an evolutionary point of view. It is not unreasonable to think that the oceans in which life arose were halophilic environments. In fact, halophilic amino acids largely coincide with some of the prebiotic molecules. Would that mean that we are extremophiles? And more interestingly, in recent explorations on Mars, they found … salt water. What if Martians were halophiles?
Source: Chemistry Biology
Gabriel Ortega is a PhD student at CIC bioGUNE Bilbao and Johns Hopkins University.